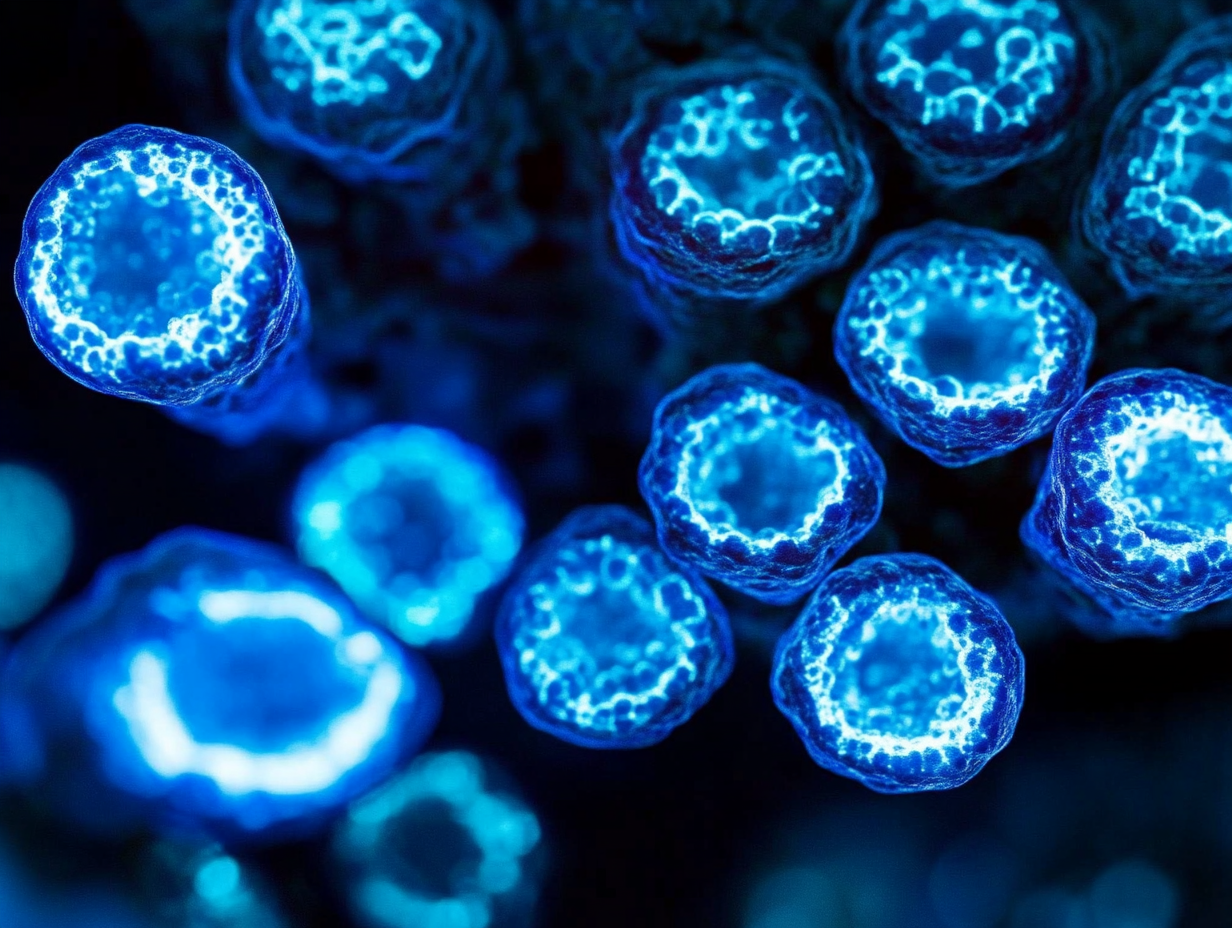
B16F10-OVA: A "Gold Standard" Model for Accelerating Cancer Immunotherapy Drug Development?
Cancer immunotherapy, including treatments like ICIs and CAR-T therapy, has revolutionized cancer treatment by activating the patient's own immune system to kill tumor cells, offering new hope [1]. However, translating these new therapies into clinical practice is a lengthy and costly process, high dependent on reliable preclinical animal models to investigate their efficacy, safety, and mechanisms of action. The B16F10-OVA model, owing to its significant advantages in evaluating antigen-specific immune responses, is extensively used in immuno-oncology research and is often regarded as a 'quasi-gold standard.'
How B16F10-OVA Aids in Immune Checkpoint Inhibitor (ICI) Research
Immune checkpoints, such as Programmed Death Receptor 1 (PD-1) and its ligand PD-L1, or Cytotoxic T-Lymphocyte-Associated protein 4 (CTLA-4), are critical "brakes" in the immune system. Under normal physiological conditions, they maintain immune homeostasis and prevent autoimmunity. However, many tumor cells hijack these pathways by overexpressing ligands like PD-L1, which bind to PD-1 on T cells, thereby inhibiting T-cell activation and effector functions, leading to immune evasion [2]. ICIs function by blocking these inhibitory signals, thus unleash the anti-tumor potential of T cells.
Empower your ICI studies with our reliable B16F10-OVA cell line. Find out more>>
The B16F10-OVA model provides an ideal in vivo platform for studying ICIs. B16F10 is a highly aggressive murine melanoma cell line derived from C57BL/6 mice, which is inherently poorly immunogenic. By genetically engineering these cells to stably express Ovalbumin (OVA), OVA serves as a well-defined, trackable tumor-associated antigen. In C57BL/6 mice engrafted with B16F10-OVA cells, researchers can:
Evaluate the anti-tumor efficacy of ICIs: By comparing tumor growth curves and survival rates between ICI-treated and control groups, the therapeutic efficacy of ICIs can be directly assessed. For instance, anti-PD-1 or anti-PD-L1 antibodies can significantly inhibit the growth of B16F10-OVA tumors.
Analyze immune cell infiltration and functional changes: Tumor tissues, draining lymph nodes, and spleens can be harvested to analyze changes in immune cell subsets, such as CD8+ T cells, CD4+ T cells, regulatory T cells (Tregs), and myeloid-derived suppressor cells (MDSCs), using techniques like flow cytometry. Crucially, the infiltration, activation status (e.g., expression of IFN-γ, Granzyme B), and proliferative capacity of OVA-specific CD8+ T cells (e.g., T cells recognizing the SIINFEKL epitope of OVA) can be measured. This provides direct insight into how ICIs enhance specific immune responses against the tumor antigen.
These analyses allow researchers not only to determine the efficacy of ICIs but also to delve into their mechanisms of action, such as whether ICIs enhance T-cell effector functions within the tumor microenvironment or alter the balance of suppressive immune cells.
A "Litmus Test" for Cancer Vaccines: Evaluating Vaccine Potency with B16F10-OVA
Cancer vaccines aim to actively prime the patient's immune system by introducing Tumor-Associated Antigens (TAAs) or Tumor-Specific Antigens (TSAs), thereby induce a durable and specific anti-tumor immune response. OVA, a highly immunogenic model antigen, functions as a TAA in the B16F10-OVA model. Its utility stems from the presence of well-characterized T-cell epitopes (e.g., the CD8+ T-cell epitope SIINFEKL and CD4+ T-cell epitopes), enabling precise tracking and quantification of antigen-specific immune responses.
A typical experimental workflow includes:
Vaccination: C57BL/6 mice are immunized with a vaccine containing OVA or encoding OVA (e.g., OVA peptides, OVA protein, OVA-expressing DNA/RNA vaccines, OVA-pulsed dendritic cell vaccines).
Tumor Challenge: After a period post-vaccination, mice are challenged with a lethal dose of B16F10-OVA cells, typically via subcutaneous or intravenous injection.
Efficacy Assessment: Tumor incidence, growth rate, and mouse survival time are monitored and recorded. An effective cancer vaccine will significantly delay tumor growth, potentially lead to complete tumor rejection, and extend survival.
Immunological Mechanism Analysis: Before or after the challenge, or at the experimental endpoint, the frequency, activation status, and cytotoxic function of OVA-specific CD8+ and CD4+ T cells are assessed (e.g., via ELISpot, intracellular cytokine staining for IFN-γ). The production of OVA-specific antibodies can also be measured.
The B16F10-OVA model empowers researchers to clearly evaluate the strength and durability of antigen-specific immunity induced by different vaccine formulations, adjuvants, delivery routes, or combination strategies, providing valuable data for vaccine design optimization.
An Ideal Proving Ground for Adoptive Cell Therapy (ACT)
Adoptive Cell Therapy (ACT), particularly CAR-T and TCR-engineered T-cell (TCR-T) therapies, represents another major leap in cancer immunotherapy. These therapies involve the ex vivo expansion and reinfusion of immune cells (primarily T cells) with anti-tumor activity to directly kill tumor cells.
The B16F10-OVA model also shines in ACT research:
Application of OT-I T cells: OT-I T cells are transgenic T cells whose T-cell receptors (TCRs) specifically recognize the SIINFEKL peptide of OVA presented by H-2Kb (the MHC class I molecule of C57BL/6 mice) [3]. Adoptive transfer of OT-I T cells into mice bearing B16F10-OVA tumors allows for direct visualization and assessment of the homing, proliferation, differentiation, and anti-tumor efficacy of these antigen-specific T cells. This platform enables researchers to optimize ex vivo T-cell activation/expansion protocols, dosing, and timing of administration.
Evaluation of novel CAR-T or TCR-T cells: If researchers are developing CAR-T cells or TCR-T cells targeting the OVA antigen, the B16F10-OVA model offers a perfect in vivo validation system. Infusion of these engineered T cells into B16F10-OVA tumor-bearing mice directly tests their ability to target and kill OVA-expressing B16F10 cells, and to assess their in vivo persistence and potential toxicities.
By monitoring tumor regression and analyzing T-cell infiltration and function within the tumor, the B16F10-OVA model provides robust support for preclinical proof-of-concept and optimization of ACT therapies.
Challenges and Considerations: The B16F10-OVA Model is Valuable, But Not a Panacea
Despite the significant advantages of the B16F10-OVA model in the aforementioned research areas, labeling it a "gold standard" might be an overstatement, as it is not without limitations:
Differences from Human Tumors: B16F10 is a murine cell line, and its biological characteristics, tumor microenvironment, and interactions with the immune system differ significantly from the complex and diverse nature of human tumors. The murine immune system, while sharing similarities with its human counterpart, also has important distinctions.
The "Special" Nature of the OVA Antigen: OVA is an exogenous, highly immunogenic antigen. The potent immune responses observed in the B16F10-OVA model may not be fully recapitulated in the clinical setting against endogenous, often less immunogenic, human tumor antigens. Human tumor antigens are typically complex, heterogeneous, and many are "self" antigens, to which the immune system may exhibit tolerance.
Model Simplicity: As a transplantable tumor model, it cannot fully mimic the complex process of human tumorigenesis, such as spontaneous tumor development, genetic heterogeneity, and long-term co-evolution with the host immune system.
Risk of Over-Optimization: Over-reliance on a single model can lead to the selection of drugs or therapies that perform exceptionally well in that specific model but fail to show efficacy in more complex or clinically relevant systems.
Therefore, when utilizing the B16F10-OVA model, researchers must be acutely aware of its strengths and limitations. Data obtained from this model, while crucial for understanding fundamental immunological principles and for the initial screening of therapeutic strategies, should be validated and complemented in more diverse and clinically relevant preclinical models, such as genetically engineered mouse models (GEMMs), patient-derived xenograft (PDX) models, and especially humanized immune system mouse models [4].
Make informed decisions in your research. Our B16F10-OVA cells provide a strong starting point. Click to learn more details.
The B16F10-OVA cell model is undoubtedly a powerful and convenient tool in cancer immunotherapy research, particularly for evaluating immune responses against a defined model antigen, screening immune checkpoint inhibitors, assessing cancer vaccine efficacy, and optimizing adoptive cell therapy strategies. However, no single preclinical model is perfect. The "gold standard" designation is perhaps more of an acknowledgment of its outstanding contributions in specific applications. To more effectively translate laboratory discoveries into clinical benefits, researchers need to judiciously select and combine multiple models, leveraging the strengths of the B16F10-OVA model while being mindful of its limitations, to more comprehensively and accurately predict the performance of novel therapies in human patients.
References
[1] Ribas, A., & Wolchok, J. D. (2018). Cancer immunotherapy using checkpoint blockade. Science, 359(6382), 1350-1355.
[2] Pardoll, D. M. (2012). The blockade of immune checkpoints in cancer immunotherapy. Nature Reviews Cancer, 12(4), 252-264.
[3] Hogquist, K. A., Jameson, S. C., Heath, W. R., Howard, J. L., Bevan, M. J., & Carbone, F. R. (1994). T cell receptor antagonist peptides induce positive selection. Cell, 76(1), 17-27.
[4] Tentler, J. J., Tan, A. C., Weekes, C. D., Jimeno, A., Leong, S., Pitts, T. M., & Eckhardt, S. G. (2012). Patient-derived tumour xenografts as models for oncology drug development. Nature Reviews Clinical Oncology, 9(6), 338-350.